CRISPR Unedited featuring Matthew Cobb (University of Manchester)
This is a machine transcription and therefore it may contain inaccuracies, errors, or mispronunciations. Notice an error you think needs changing? Please contact the Bitesize Bio team using this form: https://bit.ly/bsbtranscriptions
Welcome to CRISPR Unedited,
a bite-sized bio podcast hosted by an Anthony Adamson.
Stay on CRISPR unedited. We chat to Matthew Cobb,
professor Zoology at the University of Manchester and Popular Science writer.
We kick off at the very start of the genetic age, back in the 1970s,
The real beginning of genetic engineering, which was in,
in 1972.
And that's when the first series of papers were published, uh,
in the autumn of that year,
which enabled us for the very first time to very PRIs relatively precisely,
uh, introduced, uh, genetic material from one organism to one species.
From we move on to discuss the hot topic of scientific self-regulation and
safeguard development.
There was a, a really pioneering conference, wasn't there? Back in, was it 1975?
Yeah, early 1975. So what you gotta remember is that the, uh,
this idea of mixing up, uh,
d n a from different organisms, from bacteria,
from viruses, different kinds of viruses, uh,
had caused great con great concern.
And finally,
we end by discussing the promise of gene editing and what the future may hold.
And
Genetic engineering and gene editing may well be able to solve them in the ways
you've suggested. Um, they're equally now, uh, tomatoes on the market,
which are gonna supply, uh, particular vitamins or particular drugs. And that,
again, may be a way of getting people to accept that, you know, this has,
has been done
All this and more in this episode of CRISPR Unedited.
So, welcome to this podcast on the history of gene editing.
I'm joined today by Matthew Cobb,
who is a professor of Zoology at the University of Manchester. Matthew, uh,
thank you for joining me.
Thank you very much. Great to be here.
Uh, so this is quite a timely podcast for a couple of reasons. First of all,
Matthew, uh, you have a book out next month, I believe, on this, uh,
that relates to this very topic.
Yeah, yeah. It's, it's quite amazing. What a coincidence. Yeah. Um,
so I've got a book out, uh, in the uk. It's called The Genetic Age, uh,
our Perilous Quest to Edit Life. Uh, the book will be out in November,
uh, in the us uh, confusingly under a different title,
a rather more pompous one, which has nothing to do with me, called as God's, uh,
um, a Moral History of the Genetic Age. But anyway,
same book inside. It's got a different title and different color, but yes,
that's, that, that's why I'm here, I suspect.
Well, it sounds, sounds very dramatic in the, in the US at least. Um, and well,
the second reason as well for this timeliness of this podcast is it's been 10
years since the landmark CRISPR paper from down in chimpanzee in science, um,
which of course led to that Nobel Prize a couple years ago into 20.
And in those 10 years, we've seen the rise of that term, gene editing,
which is just everywhere these days in the scientific literature. Um,
but this term's been around a lot longer than that, hasn't it, Matthew?
Well, yeah, I mean, it's not only the, the, the 10th anniversary of, uh,
PON DA's paper.
It's the 50th anniversary of the real beginning of
genetic engineering, which was in, in 1972.
And that's when the first series of papers were published, uh,
in the autumn of that year,
which enabled us for the very first time to very pres relatively
precisely, uh, introduce, uh, genetic material from one organism,
from one species, from one whole group, or, you know, from a virus into, uh,
another organism. And that was the, the beginning of, well,
what I've called the genetic age. Everything that's happened subsequently,
including CRISPR and future methods of base editing and all the rest,
no matter what the differences in the technology, which were enormous,
they can all trace themselves back to that paper,
although series of papers in the autumn of 1972.
And what's interesting is that from the very beginning,
because this is not simply scientific work, this has always had, uh,
a practical application to it, or there's been,
there's been hovering in the background from the very beginning.
There have been concerns about safety,
about how we're going to use this technology much as there have been
over previous technologies that have been created,
great anxieties from steam engines to, you know,
the atomic power or, or whatever the,
the genetic age is what we've been living in in the last 50 years.
And it's shaped not only science, technology, medicine,
but also culture. Uh, where would the world be without Jurassic Park? Uh,
and that's, you know, so when you think of it in that way,
it's not simply a matter of a series of discoveries we've made,
but it's also about how those discoveries have course concern.
And scientists in particular have been at the forefront of actually raising
those issues. That, that's what I find particularly fascinating. Uh,
just to conclude on this, a bit of a hurrah for geneticists. You know,
'cause scientists have done all sorts of things, uh, in the past,
but genetics is the only field in
which scientists have taken a lead, said,
this experiment is very worrying.
We are not going to do it for a while until certain safety protocols
can be, uh, implemented. And it's quite extraordinary that the physicists,
for example, are working on the Manhattan Project.
They were very concerned because when the project was finally coming to
its, uh, its combination, the Germans had been defeated.
And so the whole reason why they were racing to build the atomic bomb had
disappeared. And yet it was still going to be used against Japan.
And so many of the physicists were very unhappy about that for fairly obvious
moral reasons. But they didn't actually stop work. They were open letter,
they were letters. They complained, they argued,
but they didn't actually stop work. And yet,
four times in the history of genetic engineering, uh,
in 1971, in 1974, in 2012,
and most recently in 2019,
researchers have either argued there should be a pause on research or have
actually implemented it. So I think that in that,
that's what interests not just simply amazing technology, which has, you know,
transformed all sorts of parts of our lives affected culture,
but it's also had this interaction with the, the social sphere.
And I suppose in terms of that self-regulation, um, and geneticists,
there was a, a really pioneering conference, wasn't there, back in, was it 1975?
Yeah, early 1975. So what you've gotta remember is that the, uh,
this idea of mixing up, uh,
d n a from different organisms, from bacteria,
from viruses, different kinds of viruses, uh,
had caused great con great concern that the starting point was,
was the work of Paul Berg, who won his Nobel Prize, uh, for this in 1980.
And what Berg was really interested in was trying to understand how
mammalian genes worked, which was virtually nothing was known about this.
At the beginning of the 1970s. We had some idea about bacteria,
bacterial genes in particular, things like the LAC on and so on. And so,
what he thought was, okay, if I, we understand how the LAC on works,
and we can put it, we could put, if we could put that into a mammalian cell,
into a cell line, then we could get some idea of what the cell does,
because it would have these instructions and we'd know what we'd expect it to do
and what happens. So that was quite an interesting idea.
And he was developing tools to do this lot along with a lot of other people in
Stanford. And what he also decided to,
he had a new PhD student called Janet Mertz, and he said, okay, well,
if we're gonna use, um, uh, SV 40, which is a,
a, uh, uh, a virus that causes, uh, tumors in, uh,
hamster cells. So it's, it's something that people were very interested.
'cause at the time, everybody, there's this very fashionable idea that, um,
cancer was caused by viruses, all cancers, clearly some are.
But it was the idea that, yeah, cancer is a viral disease.
And so they was trying to study SV 40, and it infects, obviously,
it infects mammalian cells. So they thought, okay, um, we can,
what would happen if we put sv, he was,
his primary interest was to use Ssv 40 to take the LAC gene and
put it into, uh, a human cell or a mammalian cell.
But he also thought for this PhD student,
why don't we do the opposite experiment?
Why don't we get the s just see what happens if we get the SV 40 potentially
cancerous, uh, gene, uh, and put it into e coli,
which is, as everybody knows, a, a gut bacterium.
And when a man called Bob Pollack, uh, cold Spring Harbor heard of this in 1971,
he said, what, you're gonna get a cancer causing gene,
and you're gonna stick it into something that lives in our gut. You are crazy.
And he basically said that to Bergen, told him to get lost,
and eventually thought about it a lot, and then said, well, actually, yeah,
it might be dangerous. And I don't really care about that experiment anyway.
It's not really what I'm interested in, so, okay, I won't do it.
So that was the first time, and it was all done in private.
Nobody knew about this. But what happened is,
with the development of Berg's method, uh,
for using phage to the phage bacterium to get the whole
of the e the, uh, LAC on from e coli,
and then put that, uh, into a, uh,
into a cell, um, very quickly,
that quite cumbersome method, which he won his Nobel Prize for what I mean,
without a matter of months, had been, uh, streamlined,
made more simple. Janet Me's PhD student was one time PhD student. Now,
uh, had turned the system from using six complicated enzymes to using two.
So people at the time said, well, you know,
any high school student could do this. I think it's a bit of an exaggeration,
but what's striking is that the innovation really got, really took off.
And within months of these papers being published 50 years ago,
there was also the realization by Stan Cohen and Herb Boyer that they could
manipulate plasmids, these weird bits of, uh,
circular d n a that you get in bacteria,
and then use those plasmids as a way of transferring between bacteria.
And that led to the idea very rapidly that, okay, you could,
you could divert anything with this technique. And when that was published,
that is what caused the initial concerns. Uh,
first in 1973 at a conference, when Bur Boyer,
who was supposed to be not saying anything, 'cause work hadn't been published,
he couldn't resist telling these young researchers,
I've done this amazing thing with Stanko. Uh, and they all went, oh my God.
You know, this is really rather alarming. And that led to the,
uh, American Association, well, the American Academy of Sciences,
national Academy of Sciences in the U S A, setting up a,
a group to think about what should happen,
how they could control this technology, what should be the rules around it.
And Berg was the, uh, put in charge of this commission.
And what they ended up with was this conference at Il Ilmar,
which is on the California coast. And, uh,
Berg chose that place because his department at Stanford regularly went up
there. So it was, it was somewhere they knew you. It was, you know, it's a,
it's conference center on the coast. Very nice.
They had this meeting about 180 scientists,
mainly from the us but from all over the world. Uh, um,
it's particularly significant, as I'll explain later on.
There were also some Russian researchers there, or Soviet researchers,
and they thrashed out over about four days in fairly heated,
uh, circumstances, a bit like a really fractious student meeting, you know,
so it was a lot of argument and toing and froing. And some of the,
they'd had this moratorium, they'd agreed in the middle of 1974, not the,
nobody would work on re what's now known as recombinant, d n a,
so d n a from more than one species. Uh, and everybody agreed, okay,
we won't do this until we know how we can do it safely. And, uh, at the meeting,
there were, the meeting was designed to re to remove the moratorium,
to come to, you know, there was no question, should we do this? It was simply,
how can we do this? So everybody, you know, as you rightly teed this up,
people praise this as an example of scientists coming to a solution,
but they didn't actually question what the technology might be used for.
And in particular, the whole debates are focused on safety. So, um,
David Baltimore, uh, later when they know very quickly, when they know the no,
very quickly won the Nobel Prize, shortly afterwards, uh, he was the chair,
and he said, right,
we're not gonna be discussing potential commercial applications.
We're not gonna be discussing environmental consequences.
We're not gonna be discussing potential use in biological warfare.
So all the kind of moral issues or political issues were taken off the agenda.
And they simply said, all we know about is bio safety.
How can we do this safely? So it's interesting that the, in fact, there were,
there, there were very detailed discussions, but they're all about, you know,
hoods and extraction and, you know, buyer safety and that, you know,
what, what p p e you gonna wear. They weren't about, Hmm,
this might do something bad. Uh, and I think that's, that,
that part of the mythology of ilr, 'cause scientists love it. 'cause hey,
we took control of this. We came up with these criteria.
We then in the US gave them to the National Institutes of Health, which said,
we won't fund anybody who doesn't use these procedures, which are basically,
you know, four, four levels, as everybody still knows, still uses, uh, of,
of biosafety. They never had the force of law. They were never put into,
they didn't bizarrely apply to the private sector.
So the private sector companies that very soon began in the
wake of, uh, the Boyer and Cohen method, uh, in particular Genentech,
which was created shortly afterwards, um,
they were completely exempt from these rules.
They generally followed them because that was the sensible thing to do.
You don't want any bad press so that after a cinema,
you get this set of rules which are gonna be applied. And there is now,
after you've had the meeting, a huge public row about it. I mean,
it becomes an immense thing all over the world where people say, oh my God,
this is terrifying. It's gonna, you know, we're all gonna die. Um,
wasn't helped by the fact that in the uk for example,
we'd had two outbreaks of smallpox, uh, from one,
from a a a, well, both from lab leaks,
one in circumstances we don't still understand. And, you know,
people had died from this. So there were, it wasn't just craziness, you know,
it was just that weird seventies thing. People were,
had legitimate reasons to be concerned about this, but amazingly,
rapidly,
rapidly when it became apparent that you could do the experiment safely,
that there people weren't gonna die of cancer or whatever.
And also, goodness me,
there was potentially an awful lot of money to be made from this by 1980,
this kind of four year crazy intense debates all over the world
about what regulations we should have just kind of evaporated. Uh,
it was also linked to political changes. You've gotta remember, you know,
all this is linked up to society. It's not just scientists or biotech geniuses.
Uh, this is when Thatcher comes to power in the uk.
It's when Reagan comes to power in the US and deregulation,
getting rid of all the, the, the red tape. Let's cut the red tape.
And so this stuff, we don't need to worry about that. Let's just let it rip.
You know, we gotta start making money. The whole global economies in the state.
These people, for example, Genentech, come up with this way of making, uh,
insulin, using, uh, using effectively the,
the human gene. It's the first time this could be done. They use the, the human,
uh, the sequence of human insulin. 'cause up until then,
you had to use animal insulins insulin, which isn't exactly the same. Uh,
you had to get it out of calves,
How to combo by as well, I imagine. Yeah,
Yeah. Well, you, well, there are a lot of calves die. So you get, you know,
it was a byproduct of the meat industry, basically. You get the pancreas,
lobb it out, send it off to the lab, but then kind of isolates it.
But now you can make this stuff in vats. And it was better in a way than the,
uh, the previous version. Not
Necessarily cheaper though.
Well, it was supposed to be, uh, but bizarre, you know,
this is one of the things. I mean,
it's because it's not simply a matter of scale, it's also, in particular,
as we know, the us it's the, uh, the way that, uh, drugs are priced.
And yeah. So we've seen this in particular,
it should be the case because it's relatively straightforward to produce that
this insulin should be, it's cheap as chips, but you know,
in particular in the US is crazy, crazy prices. I mean, and this whole, you can,
you can see, just to finish on this,
this whole period closes with the two symbols.
In 1980. On the same day, uh, Paul Berg wins his Nobel Prize,
basically for inventing genetic engineering,
or for making it turn it into a reality. And Genentech, uh,
is put on the, uh, it goes public and goes onto Wall Street.
And Herb Boyer, uh, becomes a multimillionaire Wow. Overnight, uh,
because you know, everybody, this is just before Apple, uh, goes public.
It's the first of the real excitement about biotech. Yeah,
we can a lot of speculation. Uh, and, and immense, it was the biggest launch,
most successful launch at the time that Wall Street had ever seen.
So you've got the,
the commercial wing and the academic wing kind of in, in fact,
they were on the same page of the San Fro front page of the San Francisco, uh,
Chronicle. Uh, you had Paul Berg winning his Nobel Prize, and, you know,
Genentech takes Wall Street by storm, or whatever the headline was.
I, I, I just think it's fascinating to hear the history of this,
because there'll be so many people that list this podcast that might work in a
lab. And for them, working with plasmids is just routine. You know,
they're making them day in, day out, they're companies.
And to hear the background where there was so much controversy from fear,
you know, it, it,
it's a really interesting way to view what we do on a day-to-day basis and how
things progressed over time. Yeah. And you mentioned, you know,
you know about these fears. Obviously the,
the same kind of fears have always been around about gene editing as well,
about CRISPR nine, about what are people gonna do with it?
That concept of regulation I find really interesting,
because an analogy I often use is, is, um,
if I were to go and do an experiment that involved radioactivity,
I can't order that radioactive material to my home address. I, I can't do that.
Obviously, you need to work in an institution. It's got approvals in place,
necessarily health and safety with Crispr Cas nine.
I'm not totally certain that's the case right now. Maybe it should be, because,
you know, these days companies have just, you know,
jumped on the bandwagon as well with crispr.
And there's so many commercial products out there.
It's made my life much easier as a, as a scientist.
But should we be regulating that supply that, you know,
controlling who can buy these things?
Well, there, there is a kind of regulation. So again,
this is when it all interacts with, uh, with politics again. So I said that at,
uh, at Ilmar, they didn't discuss, they wouldn't discuss, uh, genetic,
they wouldn't discuss bio weapons. Although Baltimore said,
this is potentially the most significant and terrifying application here,
but we're not gonna talk about it. One of the reasons for that is that, um, uh,
Nixon in 1969, president Nixon had just out of the blue said, okay,
we're destroying all our stocks of traditional biological weapons. I mean,
they didn't destroy all, they never do. Uh, but, you know, we're, we're,
we're not gonna, we are not gonna develop anything new.
We'll be fine just with anthrax or whatever they had developed, you know? Um,
but that meant there was no active program of developing, uh,
bio weapons in America. So I think the Americans could feel, well, actually,
you know, maybe the politicians have sorted this out.
It turns out that the five person Soviet delegation,
which was composed of, uh,
people who were kind of ridiculed by the young bucks of American molecular
genetics, there were all these old guys in their sixties, uh,
who just didn't know anything.
Three of them had been involved in a decision taken two years earlier in the
Soviet Union to develop, uh, an active bio weapons program.
Wow. And they were actively developing.
So they were there pretending to be these kind of nies who didn't understand
anything, but in fact, they knew perfectly well how this could be used. And,
uh, for complex reasons, uh,
partly because this was a way of getting money for molecular genetics in the
Soviet Union. They said to Brev, right, we will build you new bio weapons.
We will fuse, you know, new microbes, uh,
microbes and viruses together to make terrifying weapons. It took a long time,
but by, uh, the middle of the eighties, they'd actually succeeded.
And they were developing very worrying, new weapons.
All this became apparent when there were a series of defectors in the 1980s.
And, uh, eventually the collapse of the Soviet Union, uh,
the West now knew exactly what had been going on. There were books published.
I mean, I, you know, I, I just noticed that these guys were at the,
who these guys were who were at Sima. Um,
the actual groundwork of discovering all this has been done by either defectors
or by, uh, academics interest in Soviet history.
And that meant that when people now became very alarmed, because, uh,
just as with nuclear weapons, after the collapse of the Soviet Union,
there was all these bio weapons. 'cause there were,
there were thousands and thousands of researchers all over the Soviet Union
who'd been developing this either to kill people or to kill plants and animals.
There were two kind of wings to this project. So you, the West then gets very,
very alarmed about, uh, what if these, uh,
techniques fall into the wrong hands? Right? And so it could be rogue states,
terrorists. And then of course, with nine 11,
the, at the beginning of the century, uh,
the US in particular becomes absolutely terrified. There were,
it wasn't only that 9 11, 9 11 younger listeners may not remember, well, no,
nine 11 was followed virtually immediately by a series of, uh,
envelopes containing anthrax that were sent around to various people in the,
in the, in the u s a to the ca center, the, the ca capital in Washington.
And so a number of people died by in ha you know,
they opened the envelope and the spores come out.
So there's a kind of real fear about bioterrorism.
The letters were accompanied by Islamist rhetoric,
but immediately the security services, I dunno how spotted that this,
this was just kind, this, this wasn't real. It was somebody else.
Almost certainly it was a, an, uh, an American scientist who committed sign, uh,
suicide before he could be charged.
But this meant that in the US you now have this growing concern about,
uh, potential bio-terrorism.
There was the development of the first gain of function studies.
So people were starting to reconstruct or
develop viruses because they thought it was interesting. I mean,
it's first done completely by accident. They discovered that, uh,
they were trying, some Australian researchers were trying to, uh, make, uh,
mice susceptible. 'cause mice can be a terrible problem in Australia.
There are a lot of marsupials, they're invasive species.
And they were trying to find a bio weapon against the mice,
and they were working on something called mouse px.
And they discovered that they had inadvertently made a strain
of, uh, mouse px that would not be affected by,
uh, vaccines and mouse px, as the name kind of might indicate.
It's very similar to smallpox. Mm-hmm.
So these guys in Australia were absolutely terrified.
They had inadvertently stumbled upon a way of making smallpox
resistant to, uh, vaccines. So you,
you start to get the development also of the various, uh, mini pandemics of the,
the, you know, the spillover events, uh,
that are occurring in China and in the Middle East, there's Mers, there's sars.
And so the world is starting 15 years ago to start and get very antsy about
this could all be weaponized. You get the development of synthetic biology,
hacker culture, the idea that we can do this in our basement, and as you say,
you can just order stuff, you know, so journalists would order over the,
over the internet bits of the, the smallpox virus sequence,
and they just get it in the post. You know, you send it to your home.
So what the US government started to do in particular, and the UK,
is to have, uh,
systems whereby companies were supposed to be screening the sequences. You know,
what exactly are you asking? You seen, you know, a little paperclip would pop,
pop up and said, you seem to be ordering a bio weapon.
Do you really want to do this? Or, you'd get men in black and, you know,
black glasses charging into your door.
But as the example of the journalist in London shows,
you can actually order the bits and then assemble it. You know,
if you're clever, not particularly clever. I mean,
what's striking is that after the US invaded, uh, Afghanistan,
after nine 11, they, uh,
they found lots of documents written by the written by the
Al-Qaeda leadership. And one of them said, yeah,
the West keeps on saying that this bio weapon stuff is really easy.
We should try it now. They didn't succeed.
But actually it was all the rhetoric about, God, this is amazing.
This is amazingly simple. People can use this to attack us. You know,
we must control this. And the terrorists took notice, and they tried,
but it turned out to be more complicated. So yeah, there is a,
this real concern that has been going on in particular, it's been, you know,
it was nine 11 that really kind of kickstarted it, the development of the,
the recognition that there were potential new bio weapons that could be created
by this relatively straightforward technology.
And that then kind of feeds into the whole political and economic
funding of research. And research is a guided into gain of function studies.
And that in 2012 leads to them suddenly going, well, crikey,
we've now made these viruses, you know, for example, bird flu.
They'd made it, they deliberately made it transmissible through the air,
which just seems, you know, by re respiration, why on earth would you do that?
And the argument they have is, okay,
well this will enable us to understand future pandemics. Well, you know, I,
there's an argument about this, but my personal view is, well, you know,
covid shows that it didn't actually help.
No, absolutely not.
Because of how molecular genetics has helped, has enabled us to beat it.
It didn't enable us to predict what it was gonna do or in any way or form.
But there is a big argument about this, I recognize.
But the main thing is that just as in the seventies and eighties,
people who weren't trained in basic micro microbiological techniques
flooded into the area to start, you know, making new, uh,
putting human genes into microbes and creating new drugs.
The same thing happened 20 years ago,
is that lots of people could get now get funding for doing research on these mi
microbes, but didn't necessarily have the training to do so safely.
And that has been continued, the continual argument thus far.
Nothing bad has happened, but, you know, I think that's a matter of luck.
I thought that continues. I wanna move away from, you know, some, sorry. Yeah.
Als we've been talking about, obviously, um,
and talk about some of the promises, because obviously Yeah, absolutely.
We're in a, in a revolutionary era. Um, GNSS is not a new technology. And,
you know, we,
we can track the development GNS from recombinant d n A technology.
In the early nineties.
We saw Adjacents Land Max studies on Mega Nucleases first time
demonstrating that if you generate a break in the D N A within a male yourself,
then you can exploit the repair pathways that follow and make changes.
And of course, then we had, um, uh, zinc fingers, tailings,
and it was really CRISPR Cas nine that has democratized gene editing because
it's so easy to use. Where do you see the future of gene editing? Um,
because from speaking my perspective as a laboratory scientist,
it's totally revolutionized how we approach research and the things we can do
these days. But are we just still scratching the surface?
Um, well, I think there's a, as a technique for discovery,
uh, this is, I don't think you could imagine much better. It is, I mean, the,
you know, obviously people are always fiddling around with it and making the,
the, the, the guide RNAs more accurate and all the rest of it.
But as a research tool, then this is, I mean, you know,
your imagination literally is, is the limit. The problem is,
the issue comes as, as always with these things is, well,
how are you then gonna turn that into an application,
either as a reliable therapy, a reliable, uh,
technology that you could put into the fields, uh,
or as some people wish to do as a reliable technology,
you could then release into the wild,
either in terms of altering populations or, uh, you know,
de extincting, the, the, the big fantasy. Now, my, my own view is that the,
the, the devil is always in the detail. And, you know,
the science fiction of idea, okay, we can resurrect a, uh,
a mammoth fairly quickly,
kind of collapses under the weight of the actual immense technical genetic
problems there are with doing that. On the other hand, curing diseases,
I think there is, if it can be made safe, I mean, this is the problem. So,
you know, genetic gene therapy has been around since before,
um, Paul Berg's experiment.
So the earliest attempts to try and use genes to cure genetic
diseases were, were done in 1970, and then again in 1980. So these were,
they didn't work. Uh,
but these were attempts to try and solve genetic problems in,
in humans. And there have been some remarkable successes,
but the technology has been limited because, for example, uh,
with the, you know, dealing with the bubble boy disease, SS E A S E I D,
or skid, however you want to pronounce it, um,
this could be resolved,
but only by using vectors carrying the new
gene that you couldn't control the insertion of.
And this led to some patients being cured, other patients being cured,
and then developing leukemia. Because the gene had gone, you know, the, the,
the payload had been put in the wrong place and had inadvertently turned out
that the, the vector actually wanted to hit a particular target.
And that target in some, uh,
people turn out to be this gene that if mutated would cause leukemia.
So you've got this,
It's that word control, though. I mean, the word use and control.
Because when you think of the term gene editing, when you say editing something,
it's like making a deliberate change. It's, you know, you, like,
you precisely know what the outcome's gonna be.
Well, that's, that's it, yeah.
And how we have, we got the precision, I,
I would argue probably not at this point in time. Like, like you say,
it'll improve, but have we got that precision?
Well, that's the No, clearly we haven't. I mean, we haven't enough. I mean, and,
and this, this morning you've retweeted and i, I retweeted, uh,
even the apparently safer base editing techniques, which look very exciting,
could resolve, you know, single nucleotide diseases, uh,
like sickle cell disease. I mean, you know, so there are, there are,
there are tremendous potential applications here. And you just,
you read the paper and you start, you go, crikey, that, that's amazing.
This can work. You know, we could alter a single base bear, uh,
and therefore cure somebody in without getting into any worries about, uh,
germline editing, which would be done, uh, in, uh, in somatic cells.
And you'd re-inject, you know, do them ex vivo,
you'd then re-inject them and fantastic. You can now, you know, cure somebody.
And there are individuals who have gone undergone experimental
procedures. I think that's always the emphasis. This is experimental.
People have incredibly bravely,
they are literally being a Guinea pig and allowing them set their bodies to be
used to understand. But the problem is, you know, the off target effects that,
uh, I mean, I explained this to students that depending on, you know,
the ordinary crispr, depending on when it, the state of the cell cycle,
when you do it, it can have completely catastrophic effects.
Sometimes you, you can lose whole chromosomes. I recently got that.
So I talked to the students about this after our first and second year students.
And one student wrote in his response or her responses, I don't understand,
Cobb went on about off-target effects, but my lecturers never talk about that.
It just seems to be really simple, which is true. And of course,
both are true from an experimental, from a scientific point of view. I mean,
to be brutal, you know,
if your mouse has got some off-target effects and it doesn't actually affect the
junior interest, then who cares? You know? Yeah. Uh,
but if you are wanting to edit some cells that you are then gonna stick in your
body, you don't want anything, you know, to be dangerous.
So this is, I think this is,
this is the worry that we need to be absolutely confident that the techniques
we're gonna be doing, uh, using, which are potentially life transforming,
assuming that they can be delivered to everybody who needs it.
And not only to the rich.
'cause there's also a fundamental issue of health inequalities.
This absolutely highlights, we're back to the whole thing that, you know,
science and medicine don't take place in a vacuum.
They're all linked up with society. And so, you know,
who will benefit from this? How will they benefit? But if those,
uh, bio essential safety issues can be overcome,
or the risk is so low that as a society and as
individuals, we're prepared to, uh, accept those risks. Because, you know,
lots of, every time you have a general anesthetic, it's risky,
but we accept that risk. So it doesn't have to be a hundred percent,
I don't think, but people have got, we as a society have to accept those risks.
If there are any. Uh, then yeah, this can be absolutely transformational,
not just as, uh,
a way of altering various laboratory organisms or non laboratory
organisms. So, as a zoologist, you know, I was,
one of my favorite CRISPR paper from the early wave of excitement was two papers
on ants in, in cell. Now, that's the first research,
I think it's the only research paper cell has ever published on amp.
But it was astonishing because it showed that, you know, if you knew a gene, uh,
you could then manipulate it and you can deliver the, the, the,
the CRISPR constructs to the cells that you're interested in. Uh,
then you can change virtually any organism.
And that from a laboratory point of view, fantastic.
I think in terms of applications in the field, literally the fields. So,
uh, you know, one of the most astonishing, so in writing this book,
there are lots of stuff I didn't,
most of the stuff I didn't know about before I started writing it.
And the astonishing success and problems
associated with GM crops was one of the things I found most interesting. The,
the, the, the,
the research by Mary De Chilton and others who enabled
us in the eighties to be able to manipulate plants. Uh,
and then the development of, uh,
the two principle GM crops we have around the world,
one of which is bt, which enables,
makes the plant now it produces a natural insecticide.
And that has been astonishing in terms of the reduction in the amount of
insecticide that's been chucked around the planet.
So whatever your problems you may or may not have about GM crops,
that simple fact is a good thing. Uh, and that was, I mean, so Monsanto,
which no longer exists,
so I'm not a Monsanto shill because there's nobody to give me money, right? Uh,
but what I was fascinated to discover that Monsanto develops that idea,
one,
virtually as soon as the genetic engineering of plants becomes possible in
order, as they said, we've got to get out of pesticides, get outta chemicals,
they at that time argued, this is not a sustainable system.
'cause they produced, you know, uh, they produced nap arm. Uh, they,
they produced, uh, you know, synthetic grass AstroTurf, so, you know,
everything that was artificial they were doing. And as a company, they said,
right, this isn't sustainable. We've gotta change this.
We need to develop smart ways of increasing crop production. On the other hand,
they very quickly came up with the idea of, um,
also use making herbicide tolerant crops. So their poten,
their, their, uh, insects, their herbicide, gly, glyphosate,
they discovered in one of the waste pipes of one of their factories,
a bacterium that was growing there that could resist the effects of, uh,
glyphosate. So they then take the gene outta that, took that gene,
stuck it into the plants. Hey, presto, we can now spray this, uh,
herbicide everywhere. Kill all your weeds and your corn or whatever.
It will carry on growing. Fantastic. Except, you know, uh,
evolution is smarter than we are, and it's smarter than we are with b bt, uh,
crops as well. And you start to get resistance strains, et cetera, et cetera.
And so I was amazed by the ingenuity, uh,
very surprised by the initial motivation from Monsanto researchers,
and then also kind of slightly depressed when I discovered that the overall,
the consequences of all this ingenuity and technology for productivity
of crops has been no change. Yeah. So it, you know,
we have done an amazing stuff, uh, and it is astonishing,
and there are potentials there, but for the moment,
it hasn't actually transformed. And part of the reason agriculture globally,
well, it's changed it, but it hasn't transformed it.
Part of the reason for this is that the, it's all been,
all this research has been done on particular strains of wheat or
whatever, uh, you know, maze.
And those aren't necessarily the best for all circumstances.
And this is one of the reason, in a nutshell why it hasn't,
despite the dreams of some, uh,
some donors and so on hasn't transformed agriculture in Africa, because,
you know, Africa is a continent, not a country,
and has many different ecologies and systems and ways of working, uh,
and equally in China. So fine, I'll say on this, the,
the most extraordinary thing I discovered was that,
so geom crops we know 20 years ago were a real concern to people,
I think largely because of things that had nothing to do with GM crops.
So this was the epoch of the, the mad cow disease where, but nothing,
nothing to do with genetics at all. But people became worried about food.
And then there's this idea of this new food, which we're gonna use,
and this food is got some gene from another species in it,
and it's other stuff floating around in there too. Uh, and oh,
I don't like the fan, don't like the sound of that. So there's,
there's this general concern, you know, it's,
there was the mil millennial panic about all sorts of things about,
uh, bio terrorists, about GM crops. And a lot of that has faded now,
but partly because, uh, in, in Europe,
and that's still the uk despite Brexit, uh,
there are no GM crops in the immediate human food, uh, um,
chain, food chain. So we don't, we can't eat GM crops in Europe,
but we do eat animals that have been fed on GM maize or whatever.
And in America, in the us, uh, it's in the food everywhere.
And you don't know, because you're not allowed to explicitly label saying,
this was not made with GM crops. That's for some reason, you're not allowed to,
the consumers aren't given that information in the us. Um, there's
Bot technical difference here, though, isn't there,
between GM crops and gene editing crops?
Well, that is the hope. So, in the idea,
and China has been one of the main powerhouses,
this is one of the things that is really striking,
is just as China's come to dominate the world economy,
it has been the driving force in many areas of the application of genetic
engineering. It was the first country to ha to grow a GM crop. GM tobacco,
which the Americans back in the nineties said, uh, we're not gonna take this,
uh, this,
we're not gonna buy your tobacco crop because it is got gm and it might be
dangerous. Uh, there you go. Uh,
and in an indication of what has changed, China said, okay, we won't,
we won't grow it anymore. Um, that, you know, that would never happen now.
So China has been right from the outset, very interested in developing, uh,
g uh, gene therapies for humans in developing new GM crops.
But when it comes to food, when it comes to rice,
there's been a huge yeah. Argument and rouse, and I was amazed to discover that,
for example,
Greenpeace is relatively free to organize in China against GM crops.
So they have campaigns, there are demonstrations, people, you know,
and the reason for this is that it's not only the population that's not certain
about this, it's also the, the,
the Chinese army leading figures in the Chinese army. I think it's all a plot,
a western plot, to make us all sterile. So the,
the same kind of fantasies are floating about. Now, the idea is of course,
that okay, if we can gene edit, we can simply change one or two bases.
And all of the,
the apparatus that we've used to make those changes is then metabolized by
the cells and disappears. So literally,
you have got exactly the same results as you would've done by traditional
mutation, selective breeding, which is how all our crops are produced.
Then why, why should anybody complain?
Well, it's a really interesting story here about, about the humble tomato,
isn't it? Where, you know,
essentially domestication tomato to make it agriculturally, um, uh, relevant,
you know, big fruits and large plants has destroyed its taste. Yeah.
And essentially these are made by bombarding plants with radiation or
chemicals and creating like 20,000 mutations in a single generation and seeing
what sticks, what, what's good here,
and I think it was a Chinese group actually a few years ago,
publish a paper in bio technology where they went back to the wild tomato
and then rebuilt the good meat bottle, said, you know, the mutations,
the agriculture, you know, relevant mutations in the wild tomato.
And so therefore you maintain the flavor, you maintain the natural, you know,
um, uh, uh, vitamin content of the tomato, but at the same time,
you gave it those agriculturally relevant characteristics as well. So there,
it's a gray area because obviously you are only giving those tomatoes something
that's already known. Something's been tried and tested. Yeah, absolutely.
So I think, I think that's, I mean,
what that indicates is actually with, with, with agriculture,
and it's clearly that psychologically there's something odd going on here.
So let's go back to the example of, uh, insulin. You know,
everybody who has diabetes and takes insulin,
insulin is consuming a GM or using a GM product,
and they're actually injecting it into their veins.
And that doesn't seem to worry anybody. Now,
it might be because they don't know, probably don't,
might be because there's no alternative and there isn't really,
or it might be because they just assume, well, medics know best, and anyway,
this saves my life.
But there is not the same anxiety as can be found with somebody going, oh,
I'm not gonna eat this thing. It's weird. It's, you know,
Franken food or whatever.
But part of the issue with all that is actually behind in the whole
agricultural industry is that, is that it's the, it's, you know,
the GM crops we have have been designed to fit the industry.
It's not the other way around. It's the mass agriculture we've got.
It's the spraying of herbicides everywhere.
It's the use of these very fast growing big plants
that may not actually taste as much,
which have been designed to meet our desire for cheaper food. Uh,
so, you know, we are not, we're not, we as a population are not outside of this.
We're part of it, you know, and I think there are deeper problems,
and genetic engineering and gene editing may well be able to solve them in the
ways you've suggested. Um, they're equally now, uh,
tomatoes on the market which are gonna supply, uh,
particular vitamins or particular drugs. And that, again,
may be a way of getting people to accept that, you know, this has,
has been done. It is, I mean,
often the editing and scissors metaphors we use are wrong for the reasons we,
we've explained. But I think that in some of these cases,
it is actually true. It is simply the base pairs that have been altered,
and you've got same result as you would've got by random
mutation in the incredibly labor intensive ways we've been making plants for the
last 50 years, which have fed the planet, you know? Mm-hmm.
The green revolution has been extraordinary. That has, well,
Of course, we, with, uh, with climate change. Well,
there are some people saying, you know, rather than, rather than the argument,
let's slow down climate change, people are saying,
let's adapt our crops to accommodate.
Well, we're gonna have to, because, you know, things are,
the weather's changing,
so we're going to need crops that can resist desiccation, uh, you know,
periods of, uh, long periods of drought,
and there are genes around that do that.
And so I think those kind of ways, uh,
maybe a way of, yeah. I mean, it's very interesting.
The Chinese researchers who are involved in this are very insistent that there
isn't gonna be a magic bullet. And I think we've always gotta, okay,
we can all do this, and it's going to be, uh, you know, new,
new plants to meet climate change, to meet new medical needs,
or simply to increase.
Varieties are going to come about through a whole series of ways, you know,
selective breeding mutation, gene editing,
and we're gonna have to need all those different techniques. But this is,
this is very real, I think, uh, in terms of what the future holds. And it, it,
it's not kind of fantasies about making photosynthesis more effective. I mean,
you know, you, you don't need to, that may happen, but, you know,
there are more immediate ways of altering our plants to make
them resist climate change disease. It's very striking.
There's only a handful of GM crops resist disease.
It's very odd. And I, I I,
I generally don't know whether this is because it's hellishly difficult or
because there's not as much money in it. Uh, and you know, we're caught up in a,
a system which is both extraordinary. There are things I'm worried about.
I mean, we talked at the beginning about anxieties,
and what I'm fascinated by is that those anxieties keep on recurring.
Uh, I am worried about some things,
but they are to do with the edge of this technology.
Uh, and it's not GM crops. It's, uh, you know, gain of function studies,
which I'm concerned about. It's not editing a cure disease.
It's the, what we have already seen with her John Key. It's the use of,
it's the, the pointless, I would argue, uh, embryo editing,
germline editing. There's no need to do that. It's just only benefit.
Even they all now admit all the various research groups and ethical groups that
this would only benefit a few, you know, a tiny minority,
literally a few hundred people around the world could benefit from that.
And finally, that's a
Great example of self-regulation though, isn't it? Yeah, absolutely.
Obviously a terrible incident there. But straight away, the, the, uh, the,
the Royal Academy, I believe, um, um, and the national, um, um, uh,
n n I h got together post report saying this is not good. You know,
so that sort regulation came into practice.
Yeah. And the, the final element, which we haven't talked about all,
but I kind of vaguely hinted at, is the idea of gene drive.
So this is releasing self-replicating, uh,
rapidly exploding, uh, genes into pest populations,
say mosquitoes, uh, which on the one hand looks like, well,
why wouldn't you want to do that?
Because you can get rid of mosquitoes in a malaria ridden area. But, you know,
mosquitoes move. And so the, but again,
the scientists involved have been the first people to say, Hey,
this is scary. I mean, the first publication of actually showing it worked,
uh, talked to, basically used the same language of saying,
we've created a genetic bomb, which they had, uh, you know, this is a,
so I think, I don't think there's any reason to be complacent. Um,
I think there's lots of reasons to be excited, but like any technology,
uh, you've got to develop the, the,
the regulation around it. I mean, let's go back to the railways. You know,
the railways changed the world and were,
uh, both terrifying and very, you know, create great anxieties,
lots of Victorian novels about railways,
and they ended up with a system which is pretty safe. I mean,
we do have railway accidents, but as, as railway workers say that,
that the safety regulations are written in blood because there were accidents,
and so they learnt about how to make the signal safer or whatever. Um,
we've gotta avoid that. I think the genetic engineering,
we haven't had that kind of accident.
There hasn't been anything terrible has happened yet, apart from a junkie. Um,
but there's been nothing on a mass scale, and let's keep it that way.
But the way you do that is by being worried and not by dismissing concerns as,
okay, what's the same arguments as ever?
How do they apply to these new developments?
How can we ensure that there is effective regulation and also, uh,
exploitation development of new therapies, foods,
scientific discoveries, whatever it is, uh,
within a framework that we can employ safely.
Matthew, it's been absolutely fascinating. Um, I think we have to end there,
but, well, wonderful. It's been great conversation today.
Thank you so much for joining me.
Thank you very much. I, I hope listeners have found it interesting.
You've been listening to CRISPR Unedited. To access more thoughts,
help and advice on crispr,
visit bitesize bio.com/crispr-unedited.
Creators and Guests
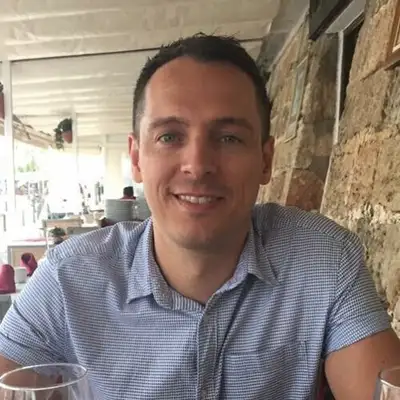
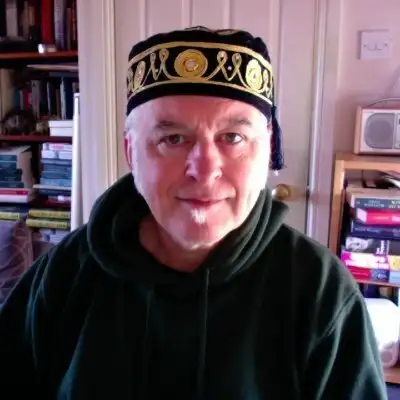
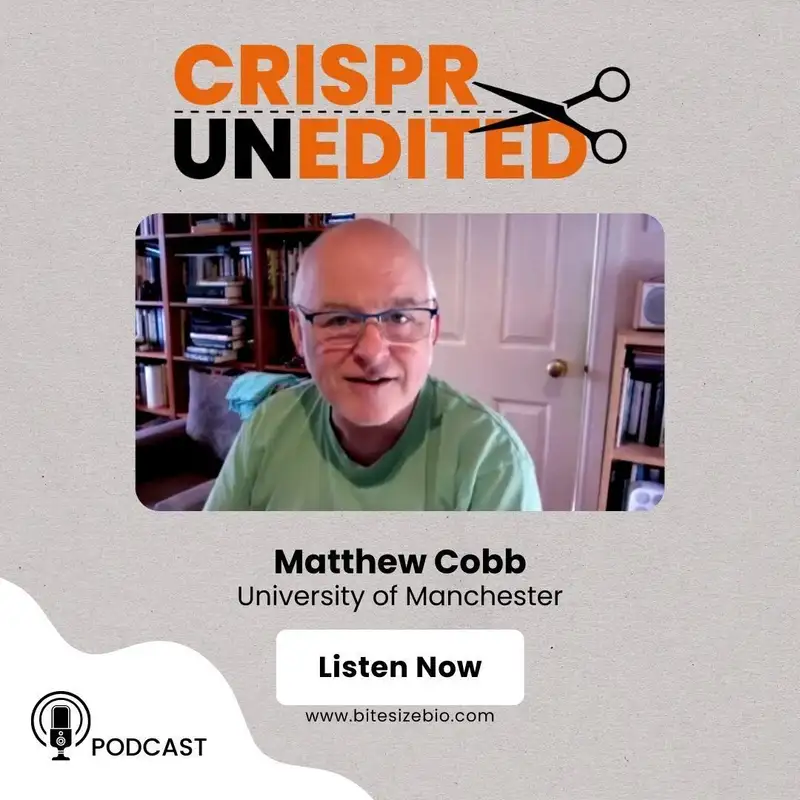